What you see is what you’ve got.
Magnetic Particle Imaging –
Conolly Lab builds the next big thing in medical imaging
Bioengineering & EECS professor Steven Conolly and his lab are a world leader in development of a new nanoparticle-based medical imaging procedure, Magnetic Particle Imaging (MPI). Over ten years into development at Berkeley, the team has recently produced groundbreaking new images and spun the technology out into a startup company, Magnetic Insight.
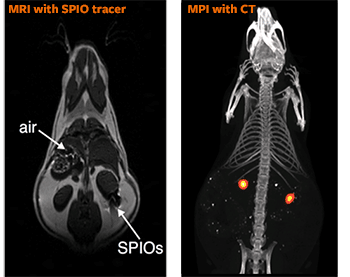
SPIO tracers block signal in MRI, showing on the image as dark spots, difficult to distinguish from naturally occurring dark spots in the body. They are also not quantitative – the spot doesn’t get more intensely dark the more cells or particles you have. In contrast, the SPIO tracers are bright and quantitative in MPI.
Although outwardly similar to Magnetic Resonance Imaging (MRI), there are several fundamental differences. Most importantly, while MRI is composed of signals that form a picture of the body – the blood, muscle, tissue – MPI does not see tissues, but creates a fully 3-dimensional image of injected tracer particles. For ease of use, MPI images are frequently layered (coregistered) with a CT, MRI, or X-ray image of the patient to show the tracer location in relation to organs or bones.
MPI uses superparamagnetic iron oxide nanoparticles (SPIOs), basically pieces of rust 10 to 30 nanometers in size, as a tracer. They respond very strongly to a magnetic field, allowing researchers to safely detect a small mass deep inside the body.
MPI images are brighter where there are greater concentrations of SPIOs, producing a truly quantitative imaging method. Mixed with other compounds, the tracer can be targeted to collect at a specific location of interest – like a tumor.
Safety and sensitivity
Techniques like nuclear medicine have a sensitivity of about 100,000 cells, or about 10,000 for MRI and 1,000 for optical imaging. Bo Zheng (PhD 2015) and collaborators have been able to improve the MPI signal-to-noise ratio from detecting deposits of 20,000 cells to imaging a group of only 200.
The group hopes to achieve resolution down to 200 picograms of tracer, or around 10 cells, but no one really knows how small it can go.
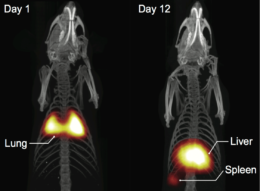
Zheng tracked injected labeled stem cells, trapped in the lungs on Day 1 and migrated to the liver by Day 12. MPI is already the most sensitive method for long-term stem cell tracking. (Theranostics, January 2016)
Because MPI uses no ionizing radiation in the scanner or tracer it is extremely safe, even for pregnant women and patients with chronic kidney disease. The SPIO tracer is well tolerated and metabolized by the liver and the spleen. Over a few months all tracer is turned into either ferritin (a natural intracellular protein) or red blood cells, or excreted.
This avoids the safety limitations of nuclear medicine, CT, and X-Ray, which use ionizing radiation, and extends the useful life of the tracer. Nuclear medicine tracers only stay visible for a short time in the body before they decay.
“MPI combines all of these advantages into one technique,” said Conolly. “It’s quantitative, it’s very sensitive, it has no depth limitations, the signal is not affected by any type of tissue, and you can look at it over a really long period of time, over months.”
Application
One of the most promising applications of MPI is in vivo stem cell tracking, a potential boon to researchers attempting to develop and deliver stem cell therapies. Optical imaging is the current state of the art in preclinical imaging, but cannot image quantitatively at significant depth inside the body. Scanners for preclinical imaging in mice and rats will be the first product of lab startup Magnetic Insight, as they continue to refine the technology.
“We want to know what happens to stem cells after we inject them into the body as therapies,” said Zheng. “There is a lack of deep imaging for tracking where stem cells go after being injected, to see if they’re cleared out by some other part of the body.”
![]() |
Tumors in dense breast tissue are obscured in X-ray and nuclear medicine screenings, compared to a Bekeley 3D MPI scan of breast cancer tumor in a rat, 6 hours after tail injection of an untargeted, long-circulating SPIO tailored for MPI by our collaborators at UW (K. Krishnan lab) and LodeSpin Labs, Seattle. (Image by Elaine Yu, UC Berkeley) |
Their recent study tracked SPIO-labeled neural progenitor cells injected directly into the frontal cortex, in collaboration with Professor Dave Schaffer’s lab. They were able to watch the cells slowly clear from the brain over a period of 87 days, a longevity impossible with nuclear medicine.
Cancer diagnosis is an application of special interest to Conolly, and MPI shows great promise for imaging tumors. The challenge is targeting them, due to the heterogeneity of tumor physiology. The lab is collaborating with about twelve different researchers on targeting cancer, an indication of the incredible challenge presented by this piece.
“Tumor detection is probably not our first application, but is our most passionate goal,” said Conolly.
“My long-term dream is to use MPI to do breast cancer screening. 47% of women have radiologically dense breast tissue, which makes X-ray mammography very difficult, but nuclear medicine studies have too much radiation to practically replace X-ray for screening. We’re trying everything we can to get a quality tumor-targeting breast scan, to create basically a non-invasive version of nuclear medicine – with no radiation.”
“If I can get that done before I retire I will be a very happy guy.”
Another early application target is cerebral perfusion imaging – post-stroke imaging to determine the size and staging of the stroke by studying how quickly blood passes through a part of the brain. With CT, the standard practice, it is easy to see where the stroke happened, but hard to tell how much of the brain is affected.
Iodine is injected and tracked, but the image contrast in capillaries between iodine and the surrounding brain structure is very low. In MPI, the scanner can see the SPIO tracers alone, with no background interference, all the way down to small capillary vessels, a huge improvement in clarity and resolution.
More immediately, they’re working on an alternative to ventilation/perfusion scans, or VQ, which are used to diagnose pulmonary embolism. There are roughly 500,000 pulmonary embolisms per year, about 1/5 of which are fatal, and they frequently recur.
CT pulmonary angiogram is most commonly used for diagnosis, but the iodine contrast agent is dangerous for people with chronic kidney disease. The alternative is nuclear medicine, using a radioactive tracer. Conolly’s lab has performed this study using MPI, with zero radiation dose, with consistent success. Second year graduate student Xinyi Zhou has made the world’s first MPI lung perfusion image using SPIO-labeled macroaggregated albumin (MAA) in rodents.
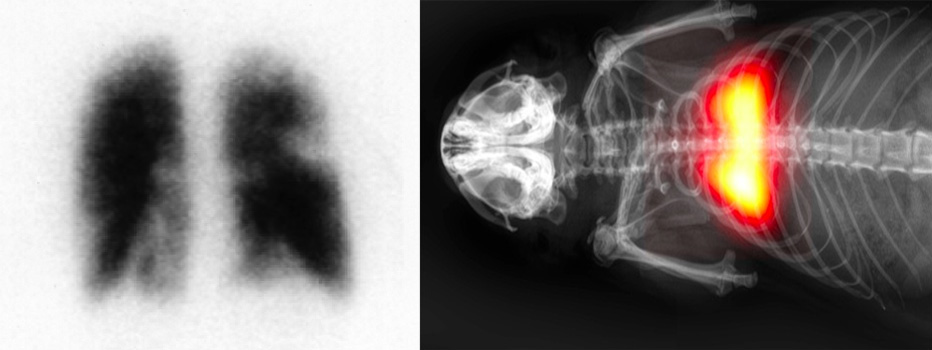
Nuclear Medicine perfusion scan for pulmonary embolism, and first MPI lung perfusion scan.
How it works
Just as MPI sees light and brightness where MRI seeks darkness near SPIOs, the scanner hardware is also inverted. An MRI scanner uses a homogeneous magnet, meaning that the magnetic field is all the same to the level of parts per billion, and applies a dynamic time-varying gradient field to generate the image contrast.
MPI does not have a rigorous need for magnet homogeneity, one of the most expensive parts of an MRI scanner. It adds a time-varying but spatially homogeneous magnetic field to a static spatially varying gradient field. MPI scans are done through xspace – the physical space as we experience it – while MRI is reconstructed through Fourier transforms. MPI is far more tolerant to variations in the magnetic field.
The patient experience will be very comparable to MRI, but will probably be faster and much less noisy, as anyone who has had an MRI will be glad to hear. Current MPI scanners are virtually silent.
From concept to market
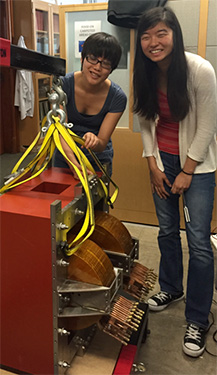
Xinyi Zhou and Elaine Yu with the core of their 6.3 Tesla/m scanner, “Helga”.
When Conolly came to Berkeley from Stanford in 2004, he was ready for a change from MRI. PhD student Patrick Goodwill’s arrival in the lab in 2006 with a background in electrical engineering and entrepreneurship coincided with a paper from Philips describing the invention of MPI, which few groups were pursuing.
“Patrick really needed to work on something big, to do something bold and different. He built basically a scanner per year by himself – which is not normal. Within only a few years he became the world’s best at building MPI scanners.”
Goodwill is now founder and CTO of Magnetic Insight. The Conolly lab has built from scratch more than six murine scanners over the last decade, including all the MPI scanners now in North America
No one has yet marketed a human MPI scanner. Conolly sees it as coming soon, within the next five years, and possibly from the Berkeley-Magnetic Insight partnership. It’s a large and expensive undertaking, but further work in the preclinical market should solve remaining optimization hurdles and reduce the costs and risk of building a human-sized scanner.